By: Justin Stone
Introduction
In baseball we hear coaches tell players to “get into your legs” or “use the ground” all the time. Coaches commonly state when describing the swing, “Hitters use the ground to create energy.”
I can’t tell you how many times players will ask, with a puzzled look: “But how?”
I want to explore the specific function of the legs and their interaction with the ground and answer this question:
What is the most important role of the legs in the swing? Is it energy and speed creation? Is it stability?
I was watching some slow motion analysis of elite discuss throwers and couldn’t help but notice the similarities to the baseball swing. Notice the following:
- Loading into the ground with force
- Counter rotation of the hips
- Explosive rotation
- Stable post leg to allow for torque and disassociation
Background Information
From our force plate studies, we know five things need to happen to create good lower body production:
- Load the rear femur
- Torque the rear femur
- Fast leg to leg transfer
- High rate of force production
- High front leg post output
The one constant that allows hitters to do all of these things is the ground: stable and unmovable.
Newton’s 3rd law is a simple one – “For every action, there is a equal and opposite reaction” (4). In terms of the baseball swing, does the force we put into the ground create a ground-reaction force that flows energy back into my legs, or is the ground simply allowing the legs to become stable, in order turn the middle of the body (hips and core), and begin kinetic energy flow?
My idea became to test swing outputs with different amounts of stability. For this I visited a gymnastics studio and swung three ways:
- Firm ground – Great stability
- Deep trampoline – Limited stability
- Suspended in air – No stability
The results and the data will paint a clearer picture on the biggest role the legs play in the swing.
When you look at the most active muscles in a baseball swing and what their anatomical role is, it’s easier to understand leg function in each phase of the swing. Referencing a 1993 EMG study by Shaffer titled, “Baseball Batting: An Electromyographic Study” we are able to correlate leg function to swing function (10). This is not intended to be an exact match to my swing or the swings posted in subsequent video analysis’, but to give the reader a better understanding of unilateral forces producing a bilateral move.
Loading Phase
Using the left handed hitter in the below video, I refer to the two sides of the body in the sagittal plane is the back side (left side for a left handed hitter) and front side (right side of a left handed hitter).
(9)
Illustrated in the video, during the loading phase, he generates over 100% of his body weight of pressure directly into the ground (Z-axis). To add, he’s counter rotating his hips and torquing his rear femur to create 48 Nm of torque. The pressure into the ground gives hitters the ability to maintain dynamic balance. This is the ability to maintain body control on one leg – in other words, stabilize the body while one foot is off of the ground.
His dynamic balance is maintained by staying connected to the ground with ample force in his back leg. He’s actively pushing into the ground while maintaining slow body control during his positive move (stride). This is demonstrated by just 3% of BW pushing reward (X-axis) during the forward advance.
Shaffer stated, during the loading phase of the swing, the posterior chain (Semimembranosus and Biceps Femoris) was leading activation with 46% and 44% of their total potential for output. Both of these muscles flex the knee (10). This is the hitter actively pushing into the ground to create stability, which bends the knee and fires the posterior chain. Notice the limitation of total hamstring output because it’s understanding is important.
The back leg loading mechanism is designed to get enough pressure into the ground to allow the body to create dynamic balance – and that is it. There is not a benefit of “getting more” into the ground that leads to “more output” into the swing. The body just needs to “get enough” force into the ground to create balance and stability on one leg during the positive advance. That body control comes from anchoring to the ground with the back foot/leg and contracting the posterior chain.
Semimembranosus
(1)
Biceps Femoris
(1)
(10)
Forward Advance
The posterior chain of the back leg gets extremely active once the stride foot is lifted in the air. Notice how EMG activity goes from 46% in the back leg load to 157% during the forward advance. To add, the biceps femoris similarly goes from 44% in the load to 154% in the advance (10). The rear leg requires more contraction to stabilize and control the body during the stride.
During this same forward advance, the body begins to create elastic stretch. On the front side of the body, the core begins to tighten, with the most active muscles being the erector spinae and the obliques. Often the torque described during the load/stride process is focused on the abdominals and obliques. Clearly from the EMG output, we see that the core is tightened completely around the body. Erector Spinae activity shows a need for more than just oblique work to stabilize the core. These muscles are extremely active.
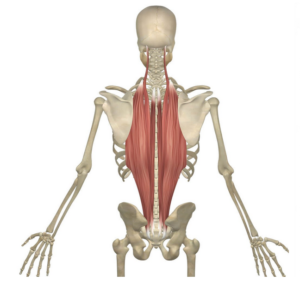
Erector Spinae (12)
(10)
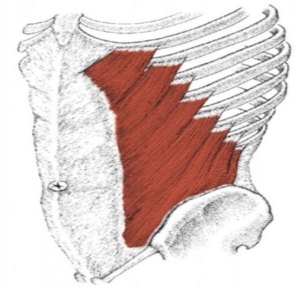
External Obliques (11)
(10)
Launch
In three baseball studies researched for EMG activity, neither Schaffer, nor a 2013 study by Nakata (7), or the 2015 study by Ohta cited the total percentage of output by the front leg during the swing (8). Therefore, I’ll reference a 2005 golf study by McHardy and Pollard titled, “Muscle Activity During the Golf Swing.” The front leg in golf acts as a stabilizer similar to the baseball swing.
As the hitter lands the vastus lateralis (the quadricep muscle on the outside of the leg) is extremely active. In the golf study, it was firing at 88% of total output (5). My hypothesis is that during the baseball swing, that would be even higher due to the forward momentum of the stride.
The vastus lateralis braces the forward momentum of the body and begins to extend the front knee through heel pressure into the ground. The adductor magnus works to help stabilize the pelvis during the turn and extend the hip. So here we have the outside of the lead leg stabilizing and helping to extend the knee and the inside of the lead thigh balancing and extending the pelvis during the turn. The adductor magnus creates an opposing force to the back glute contraction to accelerate the pelvis.
Vastus Lateralis
(3)
Adductor Magnus
(3)
On the back side of the body, the glutes are squeezing at 132%. Their peak contraction is to start the turn. The gluteal group is responsible for external hip rotation. The biceps femoris is working at 154%. The legs are contracting against one another, beginning to send that energy into a stretched-tight core (10).
Gluteus Maximus and Gluteus Medius
(3)
(10)
Acceleration to Contact
Now that stabilization has occurred on the lead leg, acceleration proceeds rapidly. This comes from the violent interaction with the front heel into the ground. Taking the knee into extension, and retracting the front hip are the large posterior chain muscles of the front leg (biceps femoris – 100% and glutes – 125%) (10). The vastus lateralis aids extension as well and provides stability. From our force plate studies, typical “good” front leg Z-force will double the back leg loading number. The high front leg post output is needed to stabilize the body and prevent a forward “slide” as well as retracting the front hip and aiding hip angular velocity during the most explosive part of the swing.
The interaction of the foot with the ground is a key component to front leg force. The heel is a direct line to the tibia and thus, the femur. Similar to squatting in the weight room, activating the posterior chain is most effective through the heel. Through testing, we find inefficient hitters often have poor or inconsistent heel interaction (getting more to the toes) and dramatically lose front leg force as a result.
Examples of Heel Interaction with the Ground
Weak Front Heel Interaction with the Ground
Notice the difference in front leg (Z-force) and the front foot/front leg interaction with the ground and “dissipation” of front leg force compared to the video below which has a violent front leg extension and “punch” of front heel into the ground.
Strong Front Heel Interaction with the Ground
Notice the spike in rate of force production with the front leg in the above video.
Acceleration to Contact Continued
The back side of the body is still using the gluteus medius to stabilize and turn the pelvis while the stretched obliques are now contracting, increasing the rotational speed of the torso (firing at 132% and 168% front and back) (10).
It’s at this point, the function of the legs is to stabilize for proper disassociation of the torso. The kinetic energy has been sent up the thorax early into acceleration of the hips. The pelvis slows its turn as the torso increases its rotational velocity. Disassociation is made easier by stability.
In this graph, the frame is stopped at the point of maximum hip acceleration. This is also the point that hip deceleration begins and torso rotational speed continues to increase. Notice how early into the turn the energy is being transferred into the core.
(10)
As illustrated in the 3D angular speed graph above, this muscle activity graph shows a similar “flow” of energy through the order of maximum contractions. Notice the highest output of the gluteus maximus is at the initiation of the turn (132%). That energy is passed to the core early into the turn when the erector spinae and obliques contract at max. From there, the role of the core is to stabilize, as energy continues to pass through the limbs and the bat.
The highest output in the swing is in both the gluteal group and the core. According to this graph and Shaffer’s study, the role of the legs is:
“The uncoiling of the wound-up pelvis, trunk, and upper extremities on a stable base provides the power of the baseball batting swing” (10).
This leads us to our study.
Testing
To test the relationship between stability and hip production in the swing, we tested in a gymnastics studio with various surface constraints.
- Firm ground – Great stability
- Deep trampoline – Limited stability
- Suspended in air – No stability
Testing on Firm Ground
My normal swing metrics on stable ground explained.
The swing on stable ground shows an efficient kinematic sequence. Notice how peak speeds of the hips, torso, lead arm and lead wrist all follow in rapid succession on the graph’s x-axis. The sequence showed my torso peaking in speed 40 milliseconds after my hips. The lead arm peaked 40 milliseconds after my torso. The wrist peaked in speed 20 milliseconds after the lead arm.
Notice how the pelvis slows faster than it accelerates, which is a sign of good stabilization and energy transfer. This is consistent with each segment in the chain – accelerating and decelerating at a faster rate than the previous segment. This shows, when locked to a stable base, the body can create better stability, as well as disassociation and stretch, thus providing greater gains in angular velocity from segment to segment.
Testing on Deep Trampoline
When you examine the graph of the swings on a deep trampoline, notice how much slower deceleration of the hips were with limited stability. The swing was still in sequence, but because hip energy was dissipated over a longer period of time, peak speeds of each segment on the x-axis were nearly on top of each other. Hips and shoulders peaked at same time. The lead arm climaxed 20 milliseconds later than the torso, at 820 deg/sec. The lead wrist then reached full speed 20 milliseconds later than the lead arm, at 969 deg/sec. The lack of separation in peak speeds on the x axis, as well as angular velocity climbs from segment to segment, shows how important stability is for disassociation. Without a stable base to turn against, the body can’t hold stretch and torque, turning more in “one-piece.” Limiting the disassociation/stretch/torque of a player, even one on the lower side of the mobility spectrum, such as myself, demonstrates a tremendous loss in angular velocity. The swing had the same sequential order as the stable swing, but produced 600 degrees per second less angular velocity.
I “felt” like my rear leg load was about the same as my normal swing because I bottomed out the trampoline, which gave me a similar feeling as the load on solid ground. Notice how the initial acceleration of the hips during the stable swing and the trampoline swing are nearly the same. The data reflects the “feeling” I had, can be confirmed. I was able to “load” my rear leg posterior chain still because the force of the back leg load was so great, it bottomed the trampoline and created back leg stability.
There was very little variability in the back leg load and ability to accelerate the hips because I was able to bottom out the trampoline with back leg Z force. 15 milliseconds difference in time to peak acceleration on stable ground and bottoming out the deep tramp is nearly identical.
The left graph below is the trampoline swing and the right graph is from the swing on stable ground.
However (and this was the biggest take away from the trampoline swing) notice how much slower deceleration occurred. The extended deceleration process was due to the time it took to stabilize my front leg during rotation. As I was creating my front leg extension, it took considerable time to bottom out the trampoline and create stability. The “dissipation,” of energy created a loss of rotational speed.
The red areas below illustrate the time from peak hip speed to complete deceleration. Left graph is the stable ground swing and the right graph is the trampoline swing.
There was a greater difference in the front leg being able to stabilize on the tramp, thus slowing deceleration ability in the hips. – 30 milliseconds of difference in deceleration times. To add, bat speed was 6 mph less on the tramp than swings with ground stabilization. Even more revealing, time to impact increased 31 milliseconds. The three metrics are verification of sheer energy and speed loss.
Testing Suspended in Air
Suspended in air, without any stability into the ground, I felt it difficult to initiate the turn. I purposely did not take any practice swings prior to the first trial (shown in the video). My body tried to use “what was available.” Without the anchor to the ground, I had a difficult time rotating my hips and core. My body still tried to stretch and contract from the middle, just without the anchor to turn against. Similar to a shortstop throwing on the run, I created stretch between my lead leg and arms in my “loading phase” and contracted my arms and lead leg against each other, while I swung the bat.
This type of cross-body, stretch-contraction, is discussed by Vleeming, as his “Anterior Oblique Sling” and similarly pointed out in the book Anatomy Trains by Thomas W. Myers, where his uniquely named myofascial meridian is, “The Spiral Line (2,6). “ Their idea, according to Myers, is “The overall movement function…is to create and mediate oblique spirals and rotations in the body, and in eccentric and isometric contraction, to steady the trunk and leg to keep it from folding into rotational collapse.”
Vleemings Anterior and Posterior Oblique Slings
(2)
Spiral Line (A myofascial meridian)
(6)
My lack of anchoring during the suspended swing created a scissor kick. Since my feet weren’t connected to the ground is was limited in creating stretch through disassociation. The lack of anchoring reduced my angular velocity of my hips to 41 deg/sec and my core had slow acceleration, reaching only 201 deg/sec.
What’s interestingly revealed: my core couldn’t decelerate and stabilize properly because of the unanchored legs. Simply stated, I was unable to disassociate without an anchor.
My arms dominated the suspended swing. My lead arm peaked in speed 20 milliseconds after the core at 618 deg/second. In the same frame, my lead wrist topped at 882 deg/sec.
The limited stabilization was from the harness holding me in the air. I felt I could, though minimally, brace my core because of the harness. The feeling was similar to that of a weight belt used in a barbell back squat. The bracing of my core allowed my arms to absorb some kinetic energy transfer.
In the suspended swing, bat path was also affected. My body was not able to hold hip hinge. When leaning forward, my legs counterbalanced by extending behind me. Without being able to tilt during the turn, my attack angle to the ball was steep compared to when my feet were on the ground. My attack angle was -28 degrees while suspended, compared to 4 or 5 degrees on swings with good or limited stabilization. Bat speed was 13 mph less than limited stability swings and 19 mph less than ground stable swings. To add, while suspended, my time to impact was 21 milliseconds slower than the limited stability swings and 52 milliseconds slower than ground stable swings.
What’s clearly apparent is that stabilization is a key component in disassociation, torque, kinetic energy transfer and speed gains.
Conclusion
Limited stability on the trampoline slowed the hip acceleration/deceleration and the amount of energy to be used further on in the kinetic chain. Torso rotational values have little difference in stable and limited stability swings. However, what differed most, was the kinetic energy built through disassociation. The hips slower rate of deceleration means the timing of the upper body turn was closer (in time) to the reduced speed, lower body turn. The body then turned in more of a “one-piece” pattern, which dramatically lowered the kinetic energy output transferred through the arms to the wrists.
Without any stability in the suspended swings, the body attempted to use the “Spiral Line” to create as much stretch-contraction energy the body had available without a leg anchor. I naturally “scissor-kicked” and blocked my front leg. Still, I was not able to disassociate and create hip-to-torso kinetic energy, since the legs were not anchored to a stable object. The core had some “limited bracing” against the harness apparatus and allowed the arms to accelerate at a similar output to the limited stability swings. The explanation of both is, disassociation was negated in both swings without ample lower body stabilization.
Though the legs aid in creating rotational hip speed, their primary function during the swing is stabilization. From the EMG study by Shaffer, he noted that the stability of the legs allows the middle of the body (glutes and core) to unwind with maximum force. The legs need to anchor to the ground, providing enough ground force to increase hip acceleration through opposing leg-to-leg forces. The bracing of the legs into the ground provides for better segment disassociation, which allows the middle of the body to stretch and turn against a stable base, thus creating better kinetic energy transfer. The ability to stabilize and disassociate allows for faster deceleration rates by the hips which increases turn speed of the torso. The increase in kinetic energy transfer promotes potential for overall, optimal, angular velocity output and bat speed.
References
1Anatomy Feed • AnatomyZone. (n.d.). Retrieved from http://anatomyzone.com/anatomy-feed/
2Anatomy Slings and Their Relationship to Low Back Pain. (n.d.). Retrieved from https://www.physio-pedia.com/Anatomy_Slings_and_Their_Relationship_to_Low_Back_Pain
3G, T. (2018, September 22). Vastus Lateralis – The Secret To Growing Huge Quads. Retrieved from https://barbellscholar.com/strength-training/vastus-lateralis-secret-growing-huge-quads/
4Gjertsen, D. (1986). The Newton handbook. London: Routledge & Kegan Paul.
5Mchardy, A. (2005). Muscle activity during the golf swing * Commentary. British Journal of Sports Medicine,39(11), 799-804. doi:10.1136/bjsm.2005.020271
6Myers, T. W. (2014). Anatomy trains: Myofascial meridans for manual and movement therapists. Edinburgh: Churchill Livingstone/Elsevier.
7Nakata, H., Miura, A., Yoshie, M., Kanosue, K., & Kudo, K. (2013). Electromyographic Analysis of Lower Limbs During Baseball Batting. Journal of Strength and Conditioning Research,27(5), 1179-1187. doi:10.1519/jsc.0b013e3182653ca9
8Ohta, Y., Nakamoto, H., Ishii, Y., Ikudome, S., Takahashi, K., & Shima, N. (2015). Muscle Activation Characteristics of the Front Leg During Baseball Swings with Timing Correction for Sudden Velocity Decrease. Plos One,10(4). doi:10.1371/journal.pone.0124113
9Quinn, M. (2015, June 11). Defending the Deadlift. Retrieved from https://fullrangecrossfit.com/defending-the-deadlift/#.XAMBbXpKjR0
10Shaffer, B., Jobe, F. W., Pink, M., & Perry, J. (1993). Baseball Batting. Clinical Orthopaedics and Related Research,&NA;(292). doi:10.1097/00003086-199307000-00038
11Stockett, S. (2018, February 28). External Obliques: Learn Your Muscles. Retrieved from https://www.custompilatesandyoga.com/external-obliques-learn-your-muscles/
12The Erector Spinae Muscles. (2018, October 22). Retrieved from https://www.yoganatomy.com/erector-spinae-muscles/